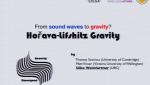
Quantum gravity without Lorentz invariance
Silke Weinfurtner University of Nottingham
Search results
Silke Weinfurtner University of Nottingham
Paul Saffin University of Nottingham
Michael Scherer Ruhr University Bochum
Gian Paolo Vacca University of Bologna
John Joseph M. Carrasco University of California, Los Angeles
Alfio Bonanno National Institute for Astrophysics (INAF)
Arkady Tseytlin Imperial College London
Omar Zanusso SISSA Scuola Internazionale Superiore di Studi Avanzati