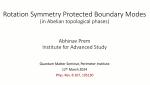
Rotation symmetry protected boundary modes in Abelian topological phases
Abhinav Prem Institute for Advanced Study (IAS)
Abhinav Prem Institute for Advanced Study (IAS)
Alexander Wilce Susquehanna University
Asimina Arvanitaki Perimeter Institute for Theoretical Physics
Mykola Semenyakin Perimeter Institute for Theoretical Physics
Eduardo Martin-Martinez University of Waterloo
Yunkai Wang Perimeter Institute for Theoretical Physics
Asimina Arvanitaki Perimeter Institute for Theoretical Physics
Jaume Gomis Perimeter Institute for Theoretical Physics
Eduardo Martin-Martinez University of Waterloo
Achim Kempf University of Waterloo