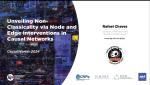
Enhancing Non-Classicality Detection with Interventions
Rafael Chaves Universidade Federal do Rio Grande do Norte
Rafael Chaves Universidade Federal do Rio Grande do Norte
Doreen Fraser University of Waterloo
Dan Wohns Perimeter Institute for Theoretical Physics
Sumati Surya Raman Research Institute
Rainer Verch Universität Leipzig
Aldo Riello Perimeter Institute for Theoretical Physics
Eric Cavalcanti Griffith University
Samuel Fedida University of Cambridge