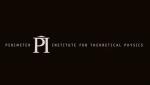
12661 - 12672 of 16889 Results
Format results
Simulation of Anyons Using Tensor Network Algorithms
Robert Pfeifer Mosgiel Health Centre
Exoplanets and the Search for Habitable Worlds
Sara Seager Massachusetts Institute of Technology (MIT) - Department of Physics
PIRSA:11010116Space-Time, Quantum Mechanics and Scattering Amplitudes
Nima Arkani-Hamed Institute for Advanced Study (IAS)