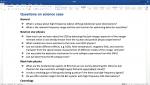
Displaying 1621 - 1632 of 5023
Format results
-
PIRSA:18060049
-
3G Detectors, Voyager
Rana Adhikari California Institute of Technology (Caltech) - Division of Physics Mathematics & Astronomy
PIRSA:18060048 -
Searching for Ultralight Particles with Gravitational Waves
Masha Baryakhtar University of Washington
PIRSA:18060047 -
Post-Merger Gravitational Wave Emission
Andreas Bauswein Max Planck Institute for Astrophysics (MPA), Garching
PIRSA:18060046 -
Hitting the High Notes: The High Frequency Dynamics of Neutron Star Mergers
William East Perimeter Institute for Theoretical Physics
PIRSA:18060045 -
-
-
Asymptotically safe gravity and electroweak scalegenesis
Masatoshi Yamada Universität Heidelberg
-
-
Asymptotically Safe Grand Unified Theories
Zhi-Wei Wang University of Waterloo
-
Keeping the Standard Model Safe
Robert Mann University of Waterloo
-