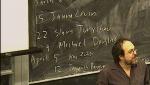
Cosmologists at Perimeter Institute seek to help pin down the constituents and history of our universe, and the rules governing its origin and evolution. Many of the most interesting clues about physics beyond the standard model (e.g., dark matter, dark energy, the matter/anti-matter asymmetry, and the spectrum of primordial density perturbations], come from cosmological observations, and cosmological observations are often the best way to test or constrain a proposed modification of the laws of nature, since such observations can probe length scales, time scales, and energy scales that are beyond the reach of terrestrial laboratories.
Displaying 2089 - 2100 of 2128
Format results
-
-
Cosmic superstrings: observable relics of brane inflation
Mark Wyman PDT Partners LLC
-
Generating scale-invaraint power spectrum in string gas cosmology
Ali Nayeri Chapman University
-
Gravity and cosmology of the dilaton at "strong coupling"
Federico Piazza Aix-Marseille University
-
-
-
-
Inflation model building and cosmic microwave background
Kenji Kadota Nagoya University
-
Dark matter and dark energy - Fact or fiction?
Philip Mannheim University of Connecticut
-
Cosmology and the AdS/CFT
Alexander Maloney McGill University
-
-
Acceleration of highest energy cosmic rays
Maxim Lyutikov Purdue University