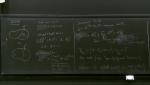
Lecture - Quantum Gravity, PHYS 644
Aldo Riello Perimeter Institute for Theoretical Physics
Search results
Aldo Riello Perimeter Institute for Theoretical Physics
Aldo Riello Perimeter Institute for Theoretical Physics
Maarten Stroeks Delft University of Technology
Jaume Gomis Perimeter Institute for Theoretical Physics
Mohamed Hibat Allah University of Waterloo
Aninda Sinha University of Calgary
William East Perimeter Institute for Theoretical Physics
Douglas Scott University of British Columbia
Alex May Perimeter Institute for Theoretical Physics
Mohamed Hibat Allah University of Waterloo
Pranav Pulakkat University of Maryland, College Park