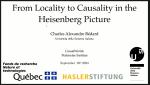
Displaying 169 - 180 of 4980
Format results
-
Charles Alexandre Bédard
-
Everything that can be learned about a causal structure with latent variables by observational and interventional probing schemes
Marina Maciel Ansanelli Perimeter Institute for Theoretical Physics
-
-
Subsystem decompositions of quantum circuits and transformations between causal perspectives
Julian Wechs Université Libre de Bruxelles
-
-
Generalized tensors and partial traces over quantum networks
Pablo Arrighi Université Paris-Sarclay and Inria
-
Emergent classicality, relativistic causality, and quantum causal structure
Nick Ormrod Perimeter Institute for Theoretical Physics
-
Which causal scenarios might support non-classical correlations?
Shashaank Khanna University of York
-
Zero Inflation as a Missing Data Problem: a Proxy-based Approach
Trung Phung Johns Hopkins Whiting School of Engineering
-
-
Relating Wigner's Friend Scenarios to Nonclassical Causal Compatibility, Monogamy Relations, and Fine Tuning
Yìlè Yīng Perimeter Institute for Theoretical Physics
-
Modeling Latent Selection with Structural Causal Models
Leihao Chen University of Amsterdam, Korteweg-de Vries Institute for Mathematics