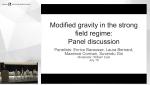
Displaying 217 - 228 of 4948
Format results
-
Enrico Barausse, Laura Bernard, Maxence Corman, Suvendu Giri
-
Formulating the complete initial boundary value problem in numerical relativity to model black hole echoes
Conner Dailey Friedrich Schiller University Jena
PIRSA:24070092 -
-
-
Assessing observational constraints on dark energy
David Shlivko Princeton University
PIRSA:24070095 -
Constructing rotating black holes in Horndeski gravity
Jibril BEN ACHOUR Arnold Sommerfeld Center (Germany) / ENS de Lyon (France)
PIRSA:24070061 -
Black hole binaries in Einstein-scalar-Gauss-Bonnet gravity and their effective-one-body description
Félix-Louis Julié Max Planck Institute for Gravitational Physics (Albert Einstein Institute)
-
Nonlinear dynamics of compact object mergers in Einstein-scalar-Gauss-Bonnet gravity
Maxence Corman Max Planck Institute for Gravitational Physics (Albert Einstein Institute)
-
Scalarized black holes - from equilibrium models to nonlinear dynamics
Daniela Doneva University of Tübingen
-
Do Neutron stars k-mouflage?
Dina Traykova Max Planck Institute for Gravitational Physics (Albert Einstein Institute)
-
Spontaneous scalarization in scalar-Gauss-Bonnet gravity and beyond
Stoytcho Yazadjiev Sofia University
PIRSA:24070090 -
Quantum Energy Conditions and Bouncing Cosmologies
Brayden Hull Perimeter Institute for Theoretical Physics
PIRSA:24070089