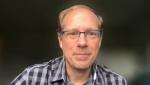
Displaying 949 - 960 of 4980
Format results
-
-
-
-
Tsung-Cheng (Peter) Lu
Tsung-Cheng Lu (Peter) University of Maryland, College Park
-
Welcome and Opening Remarks
-
Matthew Johnson York University
-
Cheng-Ju Lin University of Maryland, College Park
-
-
Good-Bye and Closing Remarks
-
Alexander Smith Saint Anselm College
-
Flaminia Giacomini ETH Zurich
-
-
Interaction and Evolution in Classical and Quantum Physics, and Indefinite Causal Structure
Erik Curiel Ludwig-Maximilians-Universität München (LMU)
-
-
Time Symmetry in Decoherence and Stable Facts
Anirban Ganguly Aix-Marseille University
-
Arrows of time and locally mediated toy-models of entanglement
Nathan Argaman Shimon Peres Negev Nuclear Research Center
-
Representing time and time's arrow
Bryan Roberts London School of Economics and Political Science
-
An experiment to detect the Discreteness of time
Marios Christodoulou University of Hong Kong (HKU)