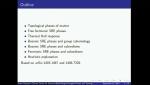
Short-Range Entangled Phases and Topology
Anton Kapustin California Institute of Technology (Caltech) - Division of Physics Mathematics & Astronomy
Anton Kapustin California Institute of Technology (Caltech) - Division of Physics Mathematics & Astronomy
Francois Huveneers University of Paris-Saclay
Bill Unruh University of British Columbia
Ralf Schuetzhold Helmholtz-Zentrum Dresden-Rossendorf
Mark Wise California Institute of Technology (Caltech)
Joshua Berger Cornell University
Martin Fluder University of Oxford
Alexander Penin University of Alberta
Senthil Todadri Massachusetts Institute of Technology (MIT) - Department of Physics
Raimar Wulkenhaar University of Münster